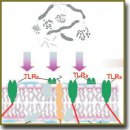
Исследование температурозависимых молекулярных механизмов развития инфекций — ключ к созданию современных профилактических средств (обзор)
Открытия в молекулярной биологии, сделанные в последние десятилетия, позволили определить ключевую роль Toll-подобных рецепторов (TLRs) в инициации врожденного и приобретенного иммунитета, в патогенезе воспалительных и аутоиммунных реакций. Возрастание клинического значения инфекций, ассоциированных с грамотрицательной флорой, и своеобразие структуры их клеточной мембраны обусловили выбор цели данного обзора — обобщение современных представлений о молекулярных механизмах развития инфекций, вызванных данной категорией микроорганизмов. В качестве актуальной модели для реализации этих молекулярных стратегий авторы рассматривают температурозависимые вариации иммуногенности патогенных видов бактерий рода Yersinia в зависимости от количества и типов ацильных групп липида А липополисахарида внешней мембраны, что оказывает модулирующее влияние на чувствительность рецептора TLR4, регулирующего иммунный ответ организма при инфекциях, которые вызваны грамотрицательными бактериями. Результаты многочисленных исследований молекулярных механизмов развития иерсиниозов и других инфекций позволили установить, что паттерны патоген-ассоциированных молекул грамотрицательных бактерий при взаимодействии с распознающими рецепторами передают множественные сигналы на клетки иммунной системы и, следовательно, могут быть использованы как природные, естественные адъюванты, активирующие адаптивный иммунный ответ организма. Эти низкомолекулярные природные адъюванты, напоминающие молекулярные компоненты липополисахарида, в настоящее время рационально использовать для конструирования современных вакцин, призванных активировать работу врожденной иммунной системы и индуцировать производство медиаторов воспаления.
- Degrave A., Siamer S., Boureau T., Barny M.A. The AvrE superfamily: ancestral type III effectors involved in suppression of pathogen-associated molecular pattern-triggered immunity. Mol Plant Pathol 2015; 16(8): 899–905, http://dx.doi.org/10.1111/mpp.12237.
- Rajamuthiah R., Mylonakis E. Effectors triggered immunity. Virulence 2014; 5(7): 697–702, http://dx.doi.org/10.4161/viru.29091.
- Park H.C., Lee S., Park B., Choi W., Kim C., Lee S., Chung W.S., Lee S.Y., Sabir J., Bressan R.A., Bohnert H.J., Mengiste T., Yun D.J. Pathogen associated molecular pattern (PAMP)-triggered immunity is compromised under C-limited growth. Mol Cells 2015; 38(1): 40–50, http://dx.doi.org/10.14348/molcells.2015.2165.
- Avdeeva M.G., Lebedev V.V., Shubich M.G. Molecular mechanisms responsible for the development of an infectious process. Klinicheskaia laboratornaia diagnostika 2007; 4: 15–22.
- Boivin A., Mesrobeanu L. Recherches sur les antigenes somatiques et sur les endotoxines des bacteries. I. Considerations generales et expose des techniques utilisees. Rev Immunol 1935; 1: 553–569.
- Rautemaa R., Meri S. Complement-resistance mechanisms of bacteria. Microbes Infect 1999; 1(10): 785–794, http://dx.doi.org/10.1016/S1286-4579(99)80081-1.
- Bliska J.B., Wang X., Viboud G.I., Brodsky I.E. Modulation of innate immune responses by Yersinia type III secretion system translocators and effectors. Cell Microbiol 2013; 15(10): 1622–1631, http://dx.doi.org/10.1111/cmi.12164.
- Avdeeva M.G., Shubich M.G. Pathogenetic mechanisms of the initiation of systemic inflammatory response syndrome (a literature review). Klinicheskaia laboratornaia diagnostika 2003; 6: 3–10.
- Matusiak M., Van Opdenbosch N., Vande Walle L., Sirard J.C., Kanneganti T.D., Lamkanfi M. Flagellin-induced NLRC4 phosphorylation primes the inflammasome for activation by NAIP5. Proc Natl Acad Sci USA 2015; 112(5): 1541–1546, http://dx.doi.org/10.1073/pnas.1417945112.
- Cornelis G.R. The type III secretion injectisome, a complex nanomachine for intracellular ‘toxin’ delivery. Biol Chem 2010; 391(7): 745–751, http://dx.doi.org/10.1515/BC.2010.079.
- Сомов Г.П., Покровский В.И., Беседнова Н.Н., Антоненко Ф.Ф. Псевдотуберкулез. М: Медицина; 2001; 256 с.
- Gioannini T.L., Teghanemt A., Zhang D., Esparza G., Yu L., Weiss J. Purified monomeric ligand. MD-2 complexes reveal molecular and structural requirements for activation and antagonism of TLR4 by Gram-negative bacterial endotoxins. Immunol Res 2014; 59(1–3): 3–11, http://dx.doi.org/10.1007/s12026-014-8543-y.
- Niu D., Wang X., Wang Y., Song X., Wang J., Guo J., Zhao H. Bacillus cereus AR156 activates PAMP-triggered immunity and induces a systemic acquired resistance through a NPR1-and SA-dependent signaling pathway. Biochem Biophys Res Commun 2016; 469(1): 120–125, http://dx.doi.org/10.1016/j.bbrc.2015.11.081.
- Boccara M., Sarazin A., Thiébeauld O., Jay F., Voinnet O., Navarro L., Colot V. Correction: the arabidopsis miR472-RDR6 silencing pathway modulates PAMP- and effector-triggered immunity through the post-transcriptional control of disease resistance genes. PLoS Pathog 2015; 11(4): e1004814, http://dx.doi.org/10.1371/journal.ppat.1004814.
- Sreekanta S., Bethke G., Hatsugai N., Tsuda K., Thao A., Wang L., Katagiri F., Glazebrook J. The receptor-like cytoplasmic kinase PCRK1 contributes to pattern-triggered immunity against Pseudomonas syringae in Arabidopsis thaliana. New Phytol 2015; 207(1): 78–90, http://dx.doi.org/10.1111/nph.13345.
- Shi Q., Febres V.J., Jones J.B., Moore G.A. Responsiveness of different citrus genotypes to the Xanthomonas citri ssp. citri-derived pathogen-associated molecular pattern (PAMP) flg22 correlates with resistance to citrus canker. Mol Plant Pathol 2015; 16(5): 507–520, http://dx.doi.org/10.1111/mpp.12206.
- Andryukov B.G., Somova L.M., Timchenko N.F. Morphological and molecular genetic markers programmed cell death of prokaryotes. Zdorov’e. Meditsinskaya ekologiya. Nauka 2015; 61(3): 4–22.
- Abolghait S.K., Iida T., Kodama T., Cantarelli V.V., Akeda Y., Honda T. Recombinant AexU effector protein of Aeromonas veronii bv. sobria disrupts the actin cytoskeleton by downregulation of Rac1 and induces direct cytotoxicity to β4-integrin expressing cell lines. Microb Pathog 2011; 51(6): 454–465, http://dx.doi.org/10.1016/j.micpath.2011.09.006.
- Pokrovskiy V.I. Immunologiya infektsionnogo protsessa [Immunology of infectious process]. Moscow: Meditsina; 1993; 360 p.
- Brubaker R.R. The recent emergence of plague: a process of felonious evolution. Microb Ecol 2004; 47(3): 293–299, http://dx.doi.org/10.1007/s00248-003-1022-y.
- Kuvacheva N.V., Morgun A.V., Hilazheva E.D., Malinovskay N.A., Gorina Y.V., Pozhilenkova E.A., Frolova O.V., Trufanova L.V., Martynova G.P., Salmina A.B. Inflammasomes forming: new mechanisms of intercellular interactions regulation and secretory activity of the cells. Sibirskoe meditsinskoe obozrenie 2013; 5: 3–10.
- Dwidar M., Leung B.M., Yaguchi T., Takayama S., Mitchell R.J. Patterning bacterial communities on epithelial cells. PLoS One 2013; 8(6): e67165, http://dx.doi.org/10.1371/journal.pone.0067165.
- Fields K.A., Nilles M.L., Cowan C., Straley S.C. Virulence role of V antigen of Yersinia pestis at the bacterial surface. Infect Immun 1999; 67(10): 5395–5408.
- Potapnev M.P. Autophagy, apoptosis, necrosis and immune recognition of self and nonself. Immunologiya 2014; 35(2): 95–102.
- Kanistanon D., Powell D.A., Hajjar A.M., Pelletier M.R., Cohen I.E., Way S.S., Skerrett S.J., Wang X., Raetz C.R., Ernst R.K. Role of Francisella lipid A phosphate modification in virulence and long-term protective immune responses. Infect Immun 2012; 80(3): 943–951, http://dx.doi.org/10.1128/IAI.06109-11.
- Raetz C.R.H. Biochemistry of endotoxins. Annu Rev Biochem 1990; 59(1): 129–170, http://dx.doi.org/10.1146/annurev.bi.59.070190.001021.
- Guo W., Zuo Z., Cheng X., Sun J., Li H., Li L., Qiu J.L. The chloride channel family gene CLCd negatively regulates pathogen-associated molecular pattern (PAMP)-triggered immunity in Arabidopsis. J Exp Bot 2014; 65(4): 1205–1215, http://dx.doi.org/10.1093/jxb/ert484.
- Quade N., Mendonca C., Herbst K., Heroven A.K., Ritter C., Heinz D.W., Dersch P. Structural basis for intrinsic thermosensing by the master virulence regulator RovA of Yersinia. J Biol Chem 2012; 287(43): 35796–35803, http://dx.doi.org/10.1074/jbc.M112.379156.
- Boller T., He S.Y. Innate immunity in plants: an arms race between pattern recognition receptors in plants and effectors in microbial pathogens. Science 2009; 324(5928): 742–744, http://dx.doi.org/10.1126/science.1171647.
- Göhre V., Jones A.M., Sklenář J., Robatzek S., Weber A.P. Molecular crosstalk between PAMP-triggered immunity and photosynthesis. Mol Plant Microbe Interact 2012; 25(8): 1083–1092, http://dx.doi.org/10.1094/MPMI-11-11-0301.
- Lewis J.D., Lo T., Bastedo P., Guttman D.S., Desveaux D. The rise of the undead: pseudokinases as mediators of effector-triggered immunity. Plant Signal Behav 2014; 9(1): e27563, http://dx.doi.org/10.4161/psb.27563.
- Medzhitov R. Toll-like receptors and innate immunity. Nat Rev Immunol 2001; 1(2): 135–145, http://dx.doi.org/10.1038/35100529.
- Medzhitov R., Preston-Hurlburt P., Janeway C.A. A human homologue of the Drosophila Toll protein signals activation of adaptive immunity. Nature 1997; 388(6640): 394–397, http://dx.doi.org/10.1038/41131.
- Matsuura M. Structural modifications of bacterial lipopolysaccharide that facilitate gram-negative bacteria evasion of host innate immunity. Front Immunol 2013; 4: 109, http://dx.doi.org/10.3389/fimmu.2013.00109.
- Krachler A.M., Woolery A.R., Orth K. Manipulation of kinase signaling by bacterial pathogens. J Cell Biol 2011; 195(7): 1083–1092, http://dx.doi.org/10.1083/jcb.201107132.
- Kurup S.P., Tarleton R.L. Perpetual expression of PAMPs necessary for optimal immune control and clearance of a persistent pathogen. Nat Commun 2013; 4: 2616, http://dx.doi.org/10.1038/ncomms3616.
- Takeuchi O., Akira S. Pattern recognition receptors and inflammation. Cell 2010; 140(6): 805–820, http://dx.doi.org/10.1016/j.cell.2010.01.022.
- Sorci G., Cornet S., Faivre B. Immune evasion, immunopathology and the regulation of the immune system. Pathogens 2013; 2(1): 71–91, http://dx.doi.org/10.3390/pathogens2010071.
- Kobayashi I., Kobayashi Y. Microtubules and pathogen defense. Plant Cell Monographs 2008; 11: 121–140, http://dx.doi.org/10.1007/7089_2007_144.
- Munford R.S. Sensing gram-negative bacterial lipopolysaccharides: a human disease determinant? Infect Immun 2008; 76(2): 454–465, http://dx.doi.org/10.1128/IAI.00939-07.
- Akopyan K., Edgren T., Wang-Edgren H., Rosqvist R., Fahlgren A., Wolf-Watz H., Fallman M. Translocation of surface-localized effectors in type III secretion. Proc Natl Acad Sci USA 2011; 108(4): 1639–1644, http://dx.doi.org/10.1073/pnas.1013888108.
- Kopp F., Kupsch S., Schromm A.B. Lipopolysaccharide-binding protein is bound and internalized by host cells and colocalizes with LPS in the cytoplasm: implications for a role of LBP in intracellular LPS-signaling. Biochim Biophys Acta 2016; 1863(4): 660–672, http://dx.doi.org/10.1016/j.bbamcr.2016.01.015.
- Stotz H.U., Mitrousia G.K., de Wit P.J., Fitt B.D. Effectors-triggered defense against apoplastic fungal pathogens. Trends Plant Sci 2014; 19(8): 491–500, http://dx.doi.org/10.1016/j.tplants.2014.04.009.
- Jensen S.B., Paludan S.R. Sensing the hybrid — a novel PAMP for TLR9. EMBO J 2014; 33(6): 529–530, http://dx.doi.org/10.1002/embj.201487747.
- Boccara M., Sarazin A., Thiébeauld O., Jay F., Voinnet O., Navarro L., Colot V. The Arabidopsis miR472-RDR6 silencing pathway modulates PAMP- and effectors-triggered immunity through the post-transcriptional control of disease resistance genes. PLoS Pathog 2014; 10(1): e1003883, http://dx.doi.org/10.1371/journal.ppat.1003883.
- Tyrer P., Foxwell A.R., Cripps A.W., Apicella M.A., Kyd J.M. Microbial pattern recognition receptors mediate M-cell uptake of a gram-negative bacterium. Infect Immun 2006; 74(1): 625–631, http://dx.doi.org/10.1128/IAI.74.1.625-631.2006.
- Raetz C.R.H., Reynolds C.M., Trent M.S., Bishop R.E. Lipid A modification systems in gram-negative bacteria. Annu Rev Biochem 2007; 76: 295–329, http://dx.doi.org/10.1146/annurev.biochem.76.010307.145803.
- Andersson J., Sjöberg O., Möller G. Mitogens as probes for immunocyte activation and cellular cooperation. Transplant Rev 1972; 11(1): 131–177, http://dx.doi.org/10.1111/j.1600-065x.1972.tb00048.x.
- Advances in inflammation research. Weissmann G. (editor). New York; 1979.
- Garcia A.V., Charrier A., Schikora A., Bigeard J., Pateyron S., de Tauzia-Moreau M.L., Evrard A., Mithöfer A., Martin-Magniette M.L., Virlogeux-Payant I., Hirt H. Salmonella enterica flagellin is recognized via FLS2 and activates PAMP-triggered immunity in Arabidopsis thaliana. Mol Plant 2014; 7(4): 657–674, http://dx.doi.org/10.1093/mp/sst145.
- Bergsbaken T., Fink S.L., Cookson B.T. Pyroptosis: host cell death and inflammation. Nat Rev Microbiol 2009; 7(2): 99–109, http://dx.doi.org/10.1038/nrmicro2070.
- Blander J.M., Sander L.E. Beyond pattern recognition: five immune checkpoints for scaling the microbial threat. Nat Rev Immunol 2012; 12(3): 215–225, http://dx.doi.org/10.1038/nri3167.
- Raetz C.R., Reynolds C.M., Trent M.S. Lipid A modification systems in gram-negative bacteria. Annu Rev Biochem 2007; 76: 295–329, http://dx.doi.org/10.1146/annurev.biochem.76.010307.145803.
- Raetz C.R.H., Whitfield C. Lipopolysaccharide endotoxins. Annu Rev Biochem 2002; 71(1): 635–700, http://dx.doi.org/10.1146/annurev.biochem.71.110601.135414.
- Matsuura M., Takahashi H., Watanabe H., Saito S., Kawahara K. Immunomodulatory effects of Yersinia pestis lipopolysaccharides on human macrophages. Clin Vaccine Immunol 2010; 17(1): 49–55, http://dx.doi.org/10.1128/CVI.00336-09.
- Matsuura M., Kawasaki K., Kawahara K., Mitsuyama M. Evasion of human innate immunity without antagonizing TLR4 by mutant Salmonella enterica serovar Typhimurium having penta-acylated lipid A. Innate Immun 2012; 18(5): 764–773, http://dx.doi.org/10.1177/1753425912440599.
- Lloyd S.R., Schoonbeek H.J., Trick M., Zipfel C., Ridout C.J. Methods to study PAMP-triggered immunity in Brassica species. Mol Plant Microbe Interact 2014; 27(3): 286–295, http://dx.doi.org/10.1094/MPMI-05-13-0154-FI.
- Kawai T., Akira S. Toll-like receptors and their crosstalk with other innate receptors in infection and immunity. Immunity 2011; 34(5): 637–650, http://dx.doi.org/10.1016/j.immuni.2011.05.006.
- Guan X., Buchholz G., Nick P. The cytoskeleton is disrupted by the bacterial effector HrpZ, but not by the bacterial PAMP flg22, in tobacco BY-2 cells. J Exp Bot 2013; 64(7): 1805–16, http://dx.doi.org/10.1093/jxb/ert042.
- Auerbuch V., Golenbock D.T., Isberg R.R. Innate immune recognition of Yersinia pseudotuberculosis type III secretion. PLoS Pathog 2009; 5(12): e1000686, http://dx.doi.org/10.1371/journal.ppat.1000686.
- Miyake K., Ogata H., Nagai Y., Akashi S., Kimoto M. Innate recognition of lipopolysaccharide by Toll-like receptor 4/MD-2 and RP105/MD-1. J Endotoxin Res 2000; 6(5): 389–391, http://dx.doi.org/10.1179/096805100101532324.
- Miyake K. Innate recognition of lipopolysaccharide by Toll-like receptor 4-MD-2. Trends Microbiol 2004; 12(4): 186–192, http://dx.doi.org/10.1016/j.tim.2004.02.009.
- Hajjar A.M., Ernst R.K., Fortuno E.S., Brasfield A.S., Yam C.S., Newlon L.A., Kollmann T.R., Miller S.I., Wilson C.B. Humanized TLR4/MD-2 mice reveal LPS recognition differentially impacts susceptibility to Yersinia pestis and Salmonella enterica. PLoS Pathog 2012; 8(10): e1002963, http://dx.doi.org/10.1371/journal.ppat.1002963.
- Takeuchi O., Kawai T., Mühlradt P.F., Morr M., Radolf J.D., Zychlinsky A., Takeda K., Akira S. Discrimination of bacterial lipoproteins by Toll-like receptor 6. Int Immunol 2001; 13(7): 933–940, http://dx.doi.org/10.1093/intimm/13.7.933.
- Zhao Q., Liu X., Zhang D., Zhang L., Luo D. Hexa-acylated LPS-lipid A deploys the appropriate level of fibrin to confer protection through MyD88. Int J Infect Dis 2015; 33: 142–148, http://dx.doi.org/10.1016/j.ijid.2015.01.021.
- Park B.S., Song D.H., Kim H.M., Choi B.S., Lee H., Lee J.O. The structural basis of lipopolysaccharide recognition by the TLR4-MD-2 complex. Nature 2009; 458(7242): 1191–1195, http://dx.doi.org/10.1038/nature07830.
- Takeuchi O., Hoshino K., Akira S. Cutting edge: TLR2-deficient and MyD88-deficient mice are highly susceptible to Staphylococcus aureus infection. J Immunol 2000; 165(10): 5392–5396, http://dx.doi.org/10.4049/jimmunol.165.10.5392.
- Maeshima N., Fernandez R.C. Recognition of lipid A variants by the TLR4-MD-2 receptor complex. Front Cell Infect Microbiol 2013; 3: 3, http://dx.doi.org/10.3389/fcimb.2013.00003.
- Tsuda K., Katagiri F. Comparing signaling mechanisms engaged in pattern-triggered and effector-triggered immunity. Curr Opin Plant Biol 2010; 13(4): 459–465, http://dx.doi.org/10.1016/j.pbi.2010.04.006.
- Liu W., Liu J., Ning Y., Ding B., Wang X., Wang Z., Wang G.L. Recent progress in understanding PAMP- and effector-triggered immunity against the rice blast fungus Magnaporthe oryzae. Mol Plant 2013; 6(3): 605–620, http://dx.doi.org/10.1093/mp/sst015.
- Gouhier-Darimont C., Schmiesing A., Bonnet C., Lassueur S., Reymond P. Signaling of Arabidopsis thaliana response to Pieris brassicae eggs shares similarities with PAMP-triggered immunity. J Exp Bot 2013; 64(2): 665–674, http://dx.doi.org/10.1093/jxb/ers362.
- Ishikawa K., Yamaguchi K., Sakamoto K., Yoshimura S., Inoue K., Tsuge S., Kojima C., Kawasaki T. Bacterial effectors modulation of host E3 ligase activity suppresses PAMP-triggered immunity in rice. Nat Commun 2014; 5: 5430–5434, http://dx.doi.org/10.1038/ncomms6430.
- Lozano-Durán R., Bourdais G., He S.Y., Robatzek S. The bacterial effector HopM1 suppresses PAMP-triggered oxidative burst and stomatal immunity. New Phytol 2014; 202(1): 259–269, http://dx.doi.org/10.1111/nph.12651.
- Kröner A., Hamelin G., Andrivon D., Val F. Quantitative resistance of potato to Pectobacterium atrosepticum and Phytophthora infestans: integrating PAMP-triggered response and pathogen growth. PLoS One 2011; 6(8): e23331, http://dx.doi.org/10.1371/journal.pone.0023331.
- Laporte J., Savin C., Lamourette P., Devilliers K., Volland H., Carniel E., Créminon C., Simon S. Fast and sensitive detection of enteropathogenic Yersinia by immunoassays. J Clin Microbiol 2015; 53(1): 146–159, http://dx.doi.org/10.1128/JCM.02137-14.
- Philip N.H., Brodsky I.E. Cell death programs in Yersinia immunity and pathogenesis. Front Cell Infect Microbiol 2012; 2: 149, http://dx.doi.org/10.3389/fcimb.2012.00149.
- Galindo C.L., Rosenzweig J.A., Kirtley M.L., Chopra A.K. Pathogenesis of Y. enterocolitica and Y. pseudotuberculosis in human yersiniosis. J Pathog 2011; 2011: e182051, http://dx.doi.org/10.4061/2011/182051.
- Auerbuch V., Golenbock D.T., Isberg R.R. Innate immune recognition of Yersinia pseudotuberculosis type III secretion. PLoS Pathog 2009; 5(12): e1000686, http://dx.doi.org/10.1371/journal.ppat.1000686.
- Bergsbaken T., Cookson B.T. Macrophage activation redirects Yersinia-infected host cell death from apoptosis to caspase-1-dependent pyroptosis. PLoS Pathog 2007; 3(11): e161, http://dx.doi.org/10.1371/journal.ppat.0030161.
- Brodsky I.E., Palm N.W., Sadanand S., Ryndak M.B., Sutterwala F.S., Flavell R.A., Bliska J.B., Medzhitov R. A Yersinia effector protein promotes virulence by preventing inflammasome recognition of the type III secretion system. Cell Host Microbe 2010; 7(5): 376–387, http://dx.doi.org/10.1016/j.chom.2010.04.009.
- Cornelis G.R. The Yersinia Ysc–Yop ‘type III’ weaponry. Nat Rev Mol Cell Biol 2002; 3(10): 742–752, http://dx.doi.org/10.1038/nrm932.
- Kawahara K., Tsukano H., Watanabe H., Lindner B., Matsuura M. Modification of the structure and activity of lipid A in Yersinia pestis lipopolysaccharide by growth temperature. Infect Immun 2002; 70(8): 4092–4098, http://dx.doi.org/10.1128/IAI.70.8.4092-4098.2002.
- Brodsky I.E., Monack D. NLR-mediated control of inflammasome assembly in the host response against bacterial pathogens. Semin Immunol 2009; 21(4): 199–207, http://dx.doi.org/10.1016/j.smim.2009.05.007.
- Du Y., Rosqvist R., Forsberg A. Role of fraction 1 antigen of Yersinia pestis in inhibition of phagocytosis. Infect Immun 2002; 70(3): 1453–1460, http://dx.doi.org/10.1128/IAI.70.3.1453-1460.2002.
- Edelmann K.H., Richardson-Burns S., Alexopoulou L., Tyler K.L., Flavell R.A., Oldstone M.B. Does Toll-like receptor 3 play a biological role in virus infections? Virology 2004; 322(2): 231–238, http://dx.doi.org/10.1016/j.virol.2004.01.033.
- Sing A., Reithmeier-Rost D., Granfors K., Hill J., Roggenkamp A., Heesemann J. A hypervariable N-terminal region of Yersinia LcrV determines Toll-like receptor 2-mediated IL-10 induction and mouse virulence. Proc Natl Acad Sci USA 2005; 102(44): 16049–16054, http://dx.doi.org/10.1073/pnas.0504728102.
- Du Y., Koh H., Park C.G., Dudziak D., Seo P., Mehandru S., Choi J.H., Cheong C., Park S., Perlin D.S., Powell B.S., Steinman R.M. Targeting of LcrV virulence protein from Yersinia pestis to dendritic cells protects mice against pneumonic plague. Eur J Immunol 2010; 40(10): 2791–2796, http://dx.doi.org/10.1002/eji.201040511.
- Saitoh S., Akashi S., Yamada T., Tanimura N., Kobayashi M., Konno K., Matsumoto F., Fukase K., Kusumoto S., Nagai Y., Kusumoto Y., Kosugi A., Miyake K. Lipid A antagonist, lipid IVa, is distinct from lipid A in interaction with Toll-like receptor 4 (TLR4)-MD-2 and ligand-induced TLR4 oligomerization. Int Immunol 2004; 16(7): 961–969, http://dx.doi.org/10.1093/intimm/dxh097.
- Jin M.S., Lee J.O. Structures of the Toll-like receptor family and its ligand complexes. Immunity 2008; 29(2): 182–191, http://dx.doi.org/10.1016/j.immuni.2008.07.007.
- Hawn T.R., Verbon A., Lettinga K.D., Zhao L.P., Li S.S., Laws R.J., Skerrett S.J., Beutler B., Schroeder L., Nachman A., Ozinsky A., Smith K.D., Aderem A. A common dominant TLR5 stop codon polymorphism abolishes flagellin signaling and is associated with susceptibility to legionnaires’ disease. J Exp Med 2003; 198(10): 1563–1572, http://dx.doi.org/10.1084/jem.20031220.
- Nagai Y., Akashi S., Nagafuku M., Ogata M., Iwakura Y., Akira S. Essential role of MD-2 in LPS responsiveness and TLR4 distribution. Nat Immunol 2002; 3(7): 667–672, http://dx.doi.org/10.1038/ni809.
- Hayashi F., Smith K.D., Ozinsky A., Hawn T.R., Yi E.C., Goodlett D.R., Eng J.K., Akira S., Underhill D.M., Aderem A. The innate immune response to bacterial flagellin is mediated by Toll-like receptor 5. Nature 2001; 410(6832): 1099–1103, http://dx.doi.org/10.1038/35074106.
- Hoshino K., Takeuchi O., Kawai T., Sanjo H., Ogawa T., Takeda Y., Takeda K., Akira S. Cutting edge: Toll-like receptor 4 (TLR4)-deficient mice are hyporesponsive to lipopolysaccharide: evidence for TLR4 as the Lps gene product. J Immunol 1999; 162(7): 3749–3752.
- Wright S.D., Ramos R.A., Tobias P.S., Ulevitch R.J., Mathison J.C. CD14, a receptor for complexes of lipopolysaccharide and LPS binding protein. Science 1990; 249(4975): 1431–1433, http://dx.doi.org/10.1126/science.1698311.
- Sing A., Roggenkamp A., Geiger A.M., Heesemann J. Yersinia enterocolitica evasion of the host innate immune response by V antigen-induced IL-10 production of macrophages is abrogated in IL-10-deficient mice. J Immunol 2002; 168(3): 1315–1321, http://dx.doi.org/10.4049/jimmunol.168.3.1315.
- Kusumoto S., Fukase K., Fukase Y., Kataoka M., Yoshizaki H., Sato K., Oikawa M., Suda Y. Structural basis for endotoxic and antagonistic activities: investigation with novel synthetic lipid A analogs. J Endotoxin Res 2003; 9(6): 361–366, http://dx.doi.org/10.1177/09680519030090060901.
- Lembo A., Pelletier M., Iyer R., Timko M., Dudda J.C., West T.E., Wilson C.B., Hajjar A.M., Skerrett S.J. Administration of a synthetic TLR4 agonist protects mice from pneumonic tularemia. J Immunol 2008; 180(11): 7574–7581, http://dx.doi.org/10.4049/jimmunol.180.11.7574.
- Dentovskaya S.V., Bakhteeva I.V., Titareva G.M., Shaikhutdinova R.Z., Anisimov A.P., Kondakova A.N., Bystrova O.V., Knirel Y.A., Lindner B. Structural diversity and endotoxic activity of the lipopolysaccharide of Yersinia pestis. Biochemistry (Moscow) 2008; 73(2): 192–199, http://dx.doi.org/10.1134/s0006297908020119.
- Schromm A.B., Brandenburg K., Loppnow H., Moran A.P., Koch M.H., Rietschel E.T., Seydel U. Biological activities of lipopolysaccharides are determined by the shape of their lipid A portion. Eur J Biochem 2000; 267(7): 2008–2013, http://dx.doi.org/10.1046/j.1432-1327.2000.01204.x.
- Schromm A.B., Lien E., Henneke P., Chow J.C., Yoshimura A., Heine H., Latz E., Monks B.G., Schwartz D.A., Miyake K., Golenbock D.T. Molecular genetic analysis of an endotoxin nonresponder mutant cell line: a point mutation in a conserved region of MD-2 abolishes endotoxin-induced signaling. J Exp Med 2001; 194(1): 79–88, http://dx.doi.org/10.1084/jem.194.1.79.
- Bergsbaken T., Cookson B.T. Innate immune response during Yersinia infection: critical modulation of cell death mechanisms through phagocyte activation. J Leukoc Biol 2009; 86(5): 1153–1158, http://dx.doi.org/10.1189/jlb.0309146.
- Rebeil R., Ernst R.K., Gowen B.B., Miller S.I., Hinnebusch B.J. Variation in lipid A structure in the pathogenic Yersiniae. Mol Microbiol 2004; 52(5): 1363–1373, http://dx.doi.org/10.1111/j.1365-2958.2004.04059.x.
- Pérez-Gutiérrez C., Llobet E., Llompart C.M., Reinés M., Bengoechea J.A. Role of lipid A acylation in Yersinia enterocolitica virulence. Infect Immun 2010; 78(6): 2768–2781, http://dx.doi.org/10.1128/IAI.01417-09.
- Perry R.D., Fetherston J.D. Yersinia pestis — etiologic agent of plague. Clin Microbiol Rev 1997; 10(1): 35–66.
- Chain P.S., Carniel E., Larimer F.W., Lamerdin J., Stoutland P.O., Regala W.M., Georgescu A.M., Vergez L.M., Land M.L., Motin V.L., Brubaker R.R., Fowler J., Hinnebusch J., Marceau M., Medigue C., Simonet M., Chenal-Francisque V., Souza B., Dacheux D., Elliott J.M., Derbise A., Hauser L.J., Garcia E. Insights into the evolution of Yersinia pestis through whole-genome comparison with Yersinia pseudotuberculosis. Proc Natl Acad Sci USA 2004; 101(38): 13826–13831, http://dx.doi.org/10.1073/pnas.0404012101.
- Montminy S.W., Khan N., McGrath S., Walkowicz M.J., Sharp F., Conlon J.E., Fukase K., Kusumoto S., Sweet C., Miyake K., Akira S., Cotter R.J., Goguen J.D., Lien E. Virulence factors of Yersinia pestis are overcome by a strong lipopolysaccharide response. Nat Immunol 2006; 7(10): 1066–1073, http://dx.doi.org/10.1038/ni1386.
- Kiljunen S., Datta N., Dentovskaya S.V., Anisimov A.P., Knirel Y.A., Bengoechea J.A., Holst O., Skurnik M. Identification of the lipopolysaccharide core of Yersinia pestis and Yersinia pseudotuberculosis as the receptor for bacteriophage φA1122. J Bacteriol 2011; 193(18): 4963–4972, http://dx.doi.org/10.1128/JB.00339-11.
- Ke Y., Chen Z., Yang R. Yersinia pestis: mechanisms of entry into and resistance to the host cell. Front Cell Infect Microbiol 2013; 3: 106, http://dx.doi.org/10.3389/fcimb.2013.00106.
- Achtman M., Zurth K., Morelli G., Torrea G., Guiyoule A., Carniel E. Yersinia pestis, the cause of plague, is a recently emerged clone of Yersinia pseudotuberculosis. Proc Natl Acad Sci USA 1999; 96(24): 14043–14048, http://dx.doi.org/10.1073/pnas.96.24.14043.
- Андрюков Б.Г., Тимченко Н.Ф. Апоптоз-модулирующие стратегии детерминант патогенности иерсиний. Здоровье. Медицинская экология. Наука 2015; 1(59): 29–41.
- Cornelis G. Yersinia type III secretion: send in the effectors. J Cell Biol 2002; 158(3): 401–408, http://dx.doi.org/10.1083/jcb.200205077.
- Kopp E., Medzhitov R. A plague on host defense. J Exp Med 2002; 196(8): 1009–1012, http://dx.doi.org/10.1084/jem.20021311.
- Kolodziejek A.M., Schnider D.R., Rohde H.N., Wojtowicz A.J., Bohach G.A., Minnich S.A., Hovde C.J. Outer membrane protein X (Ail) contributes to Yersinia pestis virulence in pneumonic plague and its activity is dependent on the lipopolysaccharide core length. Infect Immun 2010; 78(12): 5233–5243, http://dx.doi.org/10.1128/IAI.00783-10.
- Sun W., Six D.A., Reynolds C.M., Chung H.S., Raetz C.R., Curtiss R. 3rd. Pathogenicity of Yersinia pestis synthesis of 1-dephosphorylated lipid A. Infect Immun 2013; 81(4): 1172–1185, http://dx.doi.org/10.1128/IAI.01403-12.
- Knirel Y.A., Anisimov A.P. Lipopolysaccharide of Yersinia pestis, the cause of plague: structure, genetics, biological properties. Acta Naturae 2012; 4(3): 46–58.
- Reinés M., Llobet E., Dahlström K.M., Pérez-Gutiérrez C., Llompart C.M., Torrecabota N., Salminen T.A., Bengoechea J.A. Deciphering the acylation pattern of Yersinia enterocolitica lipid A. PLoS Pathog 2012; 8(10): e1002978, http://dx.doi.org/10.1371/journal.ppat.1002978.
- Ohto U., Fukase K., Miyake K., Shimizu T. Structural basis of species-specific endotoxin sensing by innate immune receptor TLR4/MD-2. Proc Natl Acad Sci USA 2012; 109(19): 7421–7426, http://dx.doi.org/10.1073/pnas.1201193109.
- Kumar H., Kawai T., Akira S. Toll-like receptors and innate immunity. Biochem Biophys Res Commun 2009; 388(4): 621–625, http://dx.doi.org/10.1016/j.bbrc.2009.08.062.
- Mikula K.M., Kolodziejczyk R., Goldman A. Yersinia infection tools-characterization of structure and function of adhesins. Front Cell Infect Microbiol 2012; 2: 169, http://dx.doi.org/10.3389/fcimb.2012.00169.
- Muszyński A., Rabsztyn K., Knapska K., Duda K.A., Duda-Grychtoł K., Kasperkiewicz K., Radziejewska-Lebrecht J., Holst O., Skurnik M. Enterobacterial common antigen and O-specific polysaccharide coexist in the lipopolysaccharide of Yersinia enterocolitica serotype O:3. Microbiology 2013; 159(Pt 8): 1782–1793, http://dx.doi.org/10.1099/mic.0.066662-0.
- Zhang Y., Bliska J.B. Role of Toll-like receptor signaling in the apoptotic response of macrophages to Yersinia infection. Infect Immun 2003; 71(3): 1513–1519, http://dx.doi.org/10.1128/IAI.71.3.1513-1519.2003.
- Qureshi S.T., Larivière L., Leveque G., Clermont S., Moore K.J., Gros P., Malo D. Endotoxin-tolerant mice have mutations in Toll-like receptor 4 (Tlr4). J Exp Med 1999; 189(4): 615–625, http://dx.doi.org/10.1084/jem.189.4.615.
- Darveau R.P. Lipid A diversity and the innate host response to bacterial infection. Curr Opin Microbiol 1998; 1(1): 36–42, http://dx.doi.org/10.1016/S1369-5274(98)80140-9.
- Kuznetsova T.A., Somova L.M., Plekhova N.G., Drobot E.I. Pathogenetic role of Yersinia pseudotuberculosis endotoxin in hemostasis and microcirculation disturbances. Bull Exp Biol Med 2011; 150(5): 619–623, http://dx.doi.org/10.1007/s10517-011-1205-3.
- Herbst K., Bujara M., Heroven A.K., Opitz W., Weichert M., Zimmermann A., Dersch P. Intrinsic thermal sensing controls proteolysis of Yersinia virulence regulator RovA. PLoS Pathog 2009; 5(5): e1000435, http://dx.doi.org/10.1371/journal.ppat.1000435.
- McNally A., Thomson N.R., Reuter S., Wren B.W. ‘Add, stir and reduce’: Yersinia spp. as model bacteria for pathogen evolution. Nat Rev Microbiol 2016; 14(3): 177–190, http://dx.doi.org/10.1038/nrmicro.2015.29.