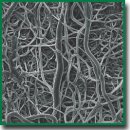
Composite Tissue-Engineered Small-Diameter Vascular Grafts Based on Polycaprolactone and Polyurethane with Growth Factors and Atrombogenic Drug Coatings: Surface Ultrastructure, Physical and Mechanical Properties
There is a growing need for synthetic small-diameter vascular grafts (<6 mm) for bypass surgery since the majority of currently developed products have demonstrated unacceptable high frequency of thrombosis in preclinical studies. The proprietary composite vascular graft based on a nonwoven polymer with anti-thrombogenic and anti-aneurysm effect and functional activity is aimed at stimulating the formation of vascular neotissue at the implantation site.
The aim of the investigation is to study the surface morphology, physical and mechanical properties of the polycaprolactone/polyurethane (PCL/PU) small-diameter tissue-engineered vascular graft with growth factors and an anti-thrombogenic surface coating.
Materials and Methods. PCL/PU vascular grafts with growth factor mix (GFmix) were manufactured using the electrospinning method. The hydrogel coating containing iloprost (Ilo) and heparin (Hep) was formed by complexation with polyvinylpyrrolidone. The controls were multilayer vascular grafts of similar composition and nonwoven matrices based on 12% PCL and 12% PU. The surface structure was analyzed with the S-3400N scanning electron microscope (Hitachi, Japan). The physical properties of the surface were determined by the wetting angle method. The mechanical properties were evaluated on a Z series universal testing machine (Zwick/Roell, Germany). Statistical processing of the data was performed using the GraphPad Prism 8 software.
Results. Our new manufacturing technique for the composite PU/PCL/GFmix/Ilo/Hep graft has eliminated the problem of graft delamination. The inner surface of the graft consisted of interwined microfibers (1.34 [1.15; 2.28] μm thick), nanofibers (790.0 [604.0; 853.5] nm thick), and interpenetrating pores of different diameters (5.4 [3.8; 8.4] μm). The process of coating formation did not affect the fibers and did not seal the pores, the surface retained its hydrophilic properties (θ=68.61±11.85°). The tensile strength (3.45 [3.17; 4.03] МPа) and Young’s modulus (4.88 [3.95; 5.80] MPa) of PU/PCL/GFmix/Ilo/Hep grafts were almost similar to the human internal thoracic artery compared to the multilayer analogs. The PU/PCL/GFmix/Ilo/Hep grafts were superior to the multilayer PCL/PU/GFmix/Ilo/Hep grafts in terms of reduced excessive elasticity (to 118.0 [111.0; 125.0]%; р=0.043).
Conclusion. The composite functionalized vascular PU/PCL/GFmix/Ilo/Hep grafts have enhanced characteristics and compliance, which, in turn, increases the probability of their high patency in future preclinical studies.
- Tinica G., Chistol R.O., Enache M., Leon Constantin M.M., Ciocoiu M., Furnica C. Long-term graft patency after coronary artery bypass grafting: effects of morphological and pathophysiological factors. Anatol J Cardiol 2018; 20(5): 275–282, https://doi.org/10.14744/AnatolJCardiol.2018.51447.
- Virani S.S., Alonso A., Benjamin E.J., Bittencourt M.S., Callaway C.W., Carson A.P., Chamberlain A.M., Chang A.R., Cheng S., Delling F.N., Djousse L., Elkind M.S.V., Ferguson J.F., Fornage M., Khan S.S., Kissela B.M., Knutson K.L., Kwan T.W., Lackland D.T., Lewis T.T., Lichtman J.H., Longenecker C.T., Loop M.S., Lutsey P.L., Martin S.S., Matsushita K., Moran A.E., Mussolino M.E., Perak A.M., Rosamond W.D., Roth G.A., Sampson U.K.A., Satou G.M., Schroeder E.B., Shah S.H., Shay C.M.,
- Spartano N.L., Stokes A., Tirschwell D.L., VanWagner L.B., Tsao C.W.; American Heart Association Council on Epidemiology and Prevention Statistics Committee and Stroke Statistics Subcommittee. Heart disease and stroke statistics — 2020 Update: a report from the American Heart Association. Circulation 2020; 141(9): e139–e596, https://doi.org/10.1161/CIR.0000000000000757.
- Pashneh-Tala S., MacNeil S., Claeyssens F. The tissue-engineered vascular graft-past, present, and future. Tissue Eng Part B Rev 2016; 22(1): 68–100, https://doi.org/10.1089/ten.teb.2015.0100.
- Lejay A., Bratu B., Kuntz S., Neumann N., Heim F., Chakfé N. Calcification of synthetic vascular grafts: a systematic review. EJVES Vasc Forum 2023; 60: 1–7, https://doi.org/10.1016/j.ejvsvf.2023.05.013.
- Yu L., Newton E.R., Gillis D.C., Sun K., Cooley B.C., Keith A.N., Sheiko S.S., Tsihlis N.D., Kibbe M.R. Coating small-diameter ePTFE vascular grafts with tunable poly(diol-co-citrate-co-ascorbate) elastomers to reduce neointimal hyperplasia. Biomater Sci 2021; 9(15): 5160–5174, https://doi.org/10.1039/d1bm00101a.
- Zhang B., Xu Y., Ma S., Wang L., Liu C., Xu W., Shi J., Qiao W., Yang H. Small-diameter polyurethane vascular graft with high strength and excellent compliance. J Mech Behav Biomed Mater 2021; 121: 104614, https://doi.org/10.1016/j.jmbbm.2021.104614. Moreno M.J., Ajji A., Mohebbi-Kalhori D., Rukhlova M., Hadjizadeh A., Bureau M.N. Development of a compliant and cytocompatible micro-fibrous polyethylene terephthalate vascular scaffold. J Biomed Mater Res B Appl Biomater 2011; 97(2): 201–214, https://doi.org/10.1002/jbm.b.31774.
- Krivkina E.O., Antonova L.V. Results of long-term patency of small-diameter biodegradable vascular prostheses with atrombogenic drug coating of sheep model. Complex Issues of Cardiovascular Diseases 2021; 10(2): 36–39, https://doi.org/10.17802/2306-1278-2021-10-2S-36-39.
- Lee K.S., Kayumov M., Emechebe G.A., Kim D.W., Cho H.J., Jeong Y.J., Lee D.W., Park J.K., Park C.H., Kim C.S., Obiweluozor F.O., Jeong I.S. A comparative study of an anti-thrombotic small-diameter vascular graft with commercially available e-PTFE graft in a porcine carotid model. Tissue Eng Regen Med 2022; 19(3): 537–551, https://doi.org/10.1007/s13770-021-00422-4.
- Lin C.H., Hsia K., Ma H., Lee H., Lu J.H. In vivo performance of decellularized vascular grafts: a review article. Int J Mol Sci 2018; 19(7): 2101, https://doi.org/10.3390/ijms19072101.
- van de Laar B.C., van Heusden H.C., Pasker-de Jong P.C., van Weel V. Omniflow II biosynthetic grafts versus expanded polytetrafluoroethylene grafts for infrainguinal bypass surgery. A single-center retrospective analysis. Vascular 2022; 30(4): 749–758, https://doi.org/10.1177/17085381211029815.
- Antonova L.V., Sevostyanova V.V., Mironov A.V., Krivkina E.O., Velikanova E.A., Matveeva V.G., Glushkova T.V., Elgudin Ya.L., Barbarash L.S. In situ vascular tissue remodeling using biodegradable tubular scaffolds with incorporated growth factors and chemoattractant molecules. Complex Issues of Cardiovascular Diseases 2018; 7(2): 25–36, https://doi.org/10.17802/2306-1278-2018-7-2-25-36.
- Tan W., Boodagh P., Selvakumar P.P., Keyser S. Strategies to counteract adverse remodeling of vascular graft: a 3D view of current graft innovations. Front Bioeng Biotechnol 2023; 10: 1097334, https://doi.org/10.3389/fbioe.2022.1097334.
- Xie X., Wu Q., Liu Y., Chen C., Chen Z., Xie C., Song M., Jiang Z., Qi X., Liu S., Tang Z., Wu Z. Vascular endothelial growth factor attenuates neointimal hyperplasia of decellularized small-diameter vascular grafts by modulating the local inflammatory response. Front Bioeng Biotechnol 2022; 10: 1066266, https://doi.org/10.3389/fbioe.2022.1066266.
- Zhang Q., Bosch-Rué È., Pérez R.A., Truskey G.A. Biofabrication of tissue engineering vascular systems. APL Bioeng 2021; 5(2): 021507, https://doi.org/10.1063/5.0039628.
- Antonova L.V., Sevostianova V.V., Silnikov V.N., Krivkina E.O., Velikanova E.A., Mironov A.V., Shabaev A.R., Senokosova E.A., Khanova M.Y., Glushkova T.V., Akentieva T.N., Sinitskaya A.V., Markova V.E., Shishkova D.K., Lobov A.A., Repkin E.A., Stepanov A.D., Kutikhin A.G., Barbarash L.S. Comparison of the patency and regenerative potential of biodegradable vascular prostheses of different polymer compositions in an ovine model. Int J Mol Sci 2023; 24(10): 8540, https://doi.org/10.3390/ijms24108540.
- Antonova L.V., Silnikov V.N., Sevostyanova V.V., Yuzhalin A.E., Koroleva L.S., Velikanova E.A., Mironov A.V., Godovikova T.S., Kutikhin A.G., Glushkova T.V., Serpokrylova I.Y., Senokosova E.A., Matveeva V.G., Khanova M.Y., Akentyeva T.N., Krivkina E.O., Kudryavtseva Y.A., Barbarash L.S. Biocompatibility of small-diameter vascular grafts in different modes of RGD modification. Polymers (Basel) 2019; 11(1): 174, https://doi.org/10.3390/polym11010174.
- Ding K., Yu X., Wang D., Wang X., Li Q. Small diameter expanded polytetrafluoroethylene vascular graft with differentiated inner and outer biomacromolecules for collaborative endothelialization, anti-thrombogenicity and anti-inflammation. Colloids Surf B Biointerfaces 2023; 229: 113449, https://doi.org/10.1016/j.colsurfb.2023.113449.
- Matsushita H., Hayashi H., Nurminsky K., Dunn T., He Y., Pitaktong I., Koda Y., Xu S., Nguyen V., Inoue T., Rodgers D., Nelson K., Johnson J., Hibino N. Novel reinforcement of corrugated nanofiber tissue-engineered vascular graft to prevent aneurysm formation for arteriovenous shunts in an ovine model. JVS Vasc Sci 2022; 3: 182–191, https://doi.org/10.1016/j.jvssci.2022.01.002.
- Siddiqui N., Asawa S., Birru B., Baadhe R., Rao S. PCL-based composite scaffold matrices for tissue engineering applications. Mol Biotechnol 2018; 60(7): 506–532, https://doi.org/10.1007/s12033-018-0084-5.
- Kucinska-Lipka J., Gubanska I., Janik H., Sienkiewicz M. Fabrication of polyurethane and polyurethane based composite fibres by the electrospinning technique for soft tissue engineering of cardiovascular system. Mater Sci Eng C Mater Biol Appl 2015; 46: 166–176, https://doi.org/10.1016/j.msec.2014.10.027.
- Tatai L., Moore T.G., Adhikari R., Malherbe F., Jayasekara R., Griffiths I., Gunatillake P.A. Thermoplastic biodegradable polyurethanes: the effect of chain extender structure on properties and in-vitro degradation. Biomaterials 2007; 28(36): 5407–5417, https://doi.org/10.1016/j.biomaterials.2007.08.035.
- Hergenrother R.W., Wabers H.D., Cooper S.L. Effect of hand segment chemistry and strain on the stability of polyurethanes: in vivo biostability. Biomaterials 1993; 14(6): 449–458, https://doi.org/10.1016/0142-9612(93)90148-u.
- Antonova L.V., Sevostyanova V.V., Mironov A.V., Krivkina E.O., Velikanova E.A., Matveeva V.G., Glushkova T.V., Elgudin Ya.L., Barbarash L.S. In situ vascular tissue remodeling using biodegradable tubular scaffolds with incorporated growth factors and chemoattractant molecules. Complex Issues of Cardiovascular Diseases 2018; 7(2): 25–36, https://doi.org/10.17802/2306-1278-2018-7-2-25-36.
- Matsuzaki Y., Miyamoto S., Miyachi H., Iwaki R., Shoji T., Blum K., Chang Y.C., Kelly J., Reinhardt J.W., Nakayama H., Breuer C.K., Shinoka T. Improvement of a novel small-diameter tissue-engineered arterial graft with heparin conjugation. Ann Thorac Surg 2021; 111(4): 1234–1241, https://doi.org/10.1016/j.athoracsur.2020.06.112.
- Antonova L.V., Sevostyanova V.V., Rezvova M.A., Krivkina E.O., Kudryavtseva Y.A., Barbarash O.L., Barbarash L.S. Technology of producing functionally active biodegradable small-diameter vascular prostheses with drug coating. Patent RU 2702239C1. 2019.
- Rickel A.P., Deng X., Engebretson D., Hong Z. Electrospun nanofiber scaffold for vascular tissue engineering. Mater Sci Eng C Mater Biol Appl 2021; 129: 112373, https://doi.org/10.1016/j.msec.2021.112373.
- Zhao L., Li X., Yang L., Sun L., Mu S., Zong H., Li Q., Wang F., Song S., Yang C., Zhao C., Chen H., Zhang R., Wang S., Dong Y., Zhang Q. Evaluation of remodeling and regeneration of electrospun PCL/fibrin vascular grafts in vivo. Mater Sci Eng C Mater Biol Appl 2021; 118: 111441, https://doi.org/10.1016/j.msec.2020.111441.
- Antonova L.V., Krivkina E.O., Sevostianova V.V., Mironov A.V., Rezvova M.A., Shabaev A.R., Tkachenko V.O., Krutitskiy S.S., Khanova M.Y., Sergeeva T.Y., Matveeva V.G., Glushkova T.V., Kutikhin A.G., Mukhamadiyarov R.A., Deeva N.S., Akentieva T.N., Sinitsky M.Y., Velikanova E.A., Barbarash L.S. Tissue-engineered carotid artery interposition grafts demonstrate high primary patency and promote vascular tissue regeneration in the ovine model. Polymers (Basel) 2021; 13(16): 2637, https://doi.org/10.3390/polym13162637.
- Antonova L.V., Krivkina E.O., Silnikov V.N., Gruzdeva O.V., Rezvova M.A., Akentieva T.N., Glushkova T.V., Tkachenko V.O., Sakharova V.M., Barbarash L.S. Evaluation of the biocompatibility and antimicrobial properties of biodegradable vascular grafts of various polymer composition with atrombogenic and antimicrobial drug coating. Vestnik transplantologii i iskusstvennyh organov 2021; 23(2): 122–136, https://doi.org/10.15825/1995-1191-2021-2-122-136.